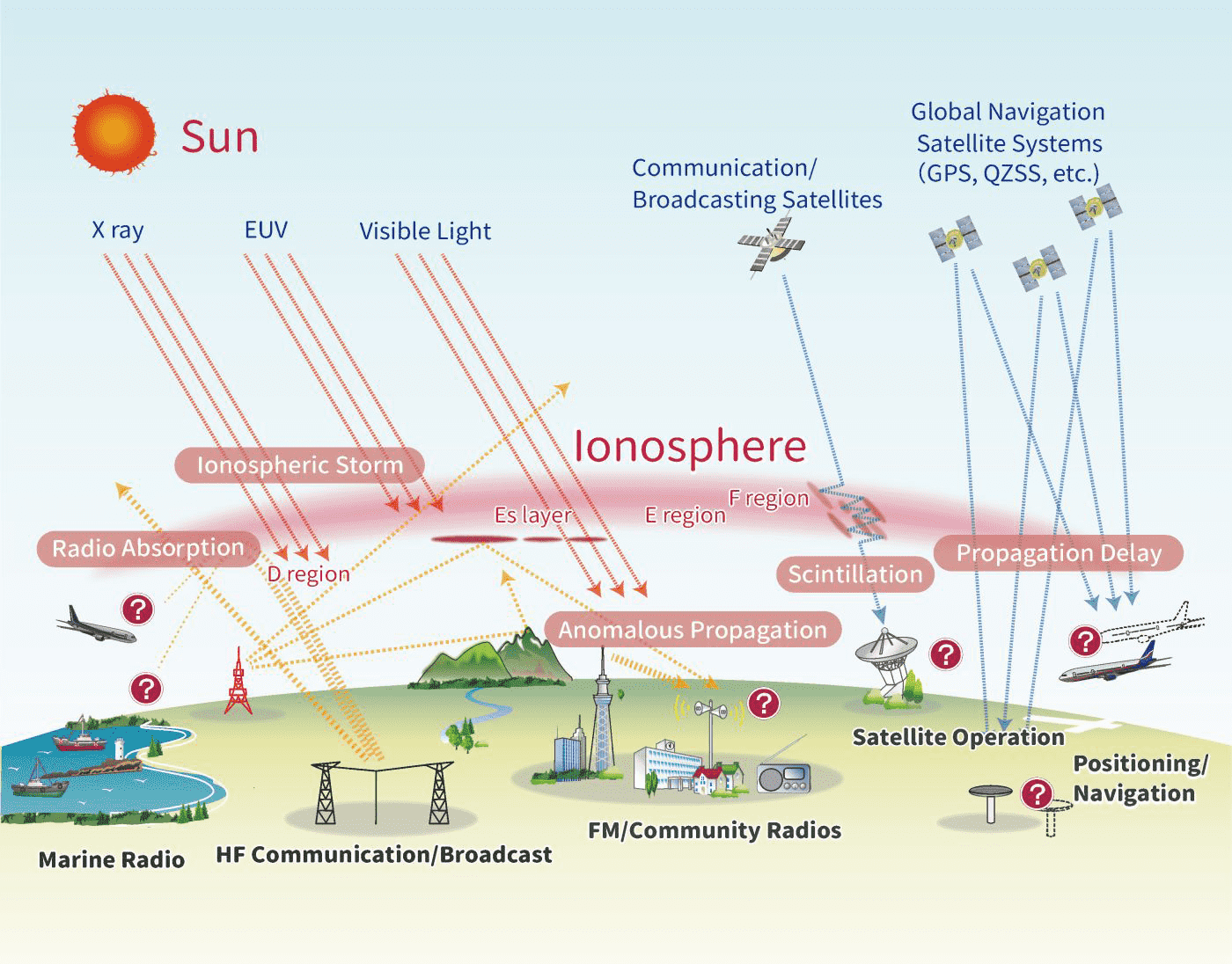
Radio waves at medium frequency (MF, 300 kHz – 3 MHz) and high frequency (HF, 3 – 30 MHz) are reflected at the ionosphere, and they have been used as a over-the-horizon communication method for a long time. Today, MF and HF are used in aviation communication, marine radio communication, broadcasting, and so forth. Such radio communications are affected by ionospheric disturbances. Immediately after the occurrence of a solar flare, strong X-rays from the sun cause abnormal ionization in the ionospheric D region in the dayside hemisphere. In this condition, radio waves at MHz frequency or lower are absorbed in the D region, resulting in communication failure. After intense solar flares, similar communication problems occur in the polar D region owing to high-energy solar protons from the sun. During a negative ionospheric storm, in the frequency range of MHz to 10 MHz will not be reflected at the ionosphere, therefore it cannot be used for long-distance communications. Furthermore, when the sporadic- E (Es) layer develops, radio waves at 10 MHz order, which normally penetrate the ionosphere, will be reflected at the Es layer, and propagate anomalously beyond the line of sight. This condition affect the long distance radio broadcasting and the wireless emergency alerts. Radio waves at higher frequency which generally penetrate the ionosphere are also affected. In the irregular conditions of ionosphere such as the plasma bubbles, which generated in the equatorial region and sometimes extend to the mid latitude region, cause fluctuations in the strength and phase of the GHz-band radio waves used for communication/broadcasting satellites, remote sensing satellites, global navigation satellites, and so forth. This phenomenon is called ionospheric scintillation, and causes the loss of lock on satellite signals in the worst case.